The short answer is: Yes, in principle. When always using the median of crop yields across the entire ensemble of crop models participating in the ISIMIP2a GGCMI model evaluation exercise, the derived drop of these median yields at high temperatures is close to the observed one. The study supports the hypothesis that the observed declining yields due to high temperatures are actually induced by water deficits caused by high temperatures as they mostly disappear under irrigations - in the observations as well as in the simulations.
Responses of individual models are still quite different and not necessarily in line with the observations. There is still some work to understand these individual results. But let’s start from the beginning…
Why did we want to check?
Our study was triggered by a famous study by US colleagues around Wolfgang Schlenker who used a huge number of crop yield observations at relative high spatial resolution (US county level) collected from 1950-2005 (Schlenker and Roberts 2009). Their results were published in 2017 and demonstrate that maize, soy and cotton show a strong drop in yields when exposed to high temperatures above 30°C (or slightly higher for cotton, 32°C) during their growing seasons. The study was interesting from a purely technical point of view as the author managed to estimate the yield effects of exposure to different temperature levels from only knowing reported yields on county level for a large number of growing seasons. But of course you also get worried if you know the climate projections and the strong rise in temperatures that this may imply depending on our greenhouse gas emissions. So we wanted to test whether the effect is included in the crop models participating in ISIMIP to make sure that we do not miss a critical process when using them for future yield projections accounting for climate change.
What do we know about the effects of temperatures?
High temperatures can directly damage the plant’s cells and impair flowering among various other effects. In addition, there is the indirect effect of high temperatures on water supply and demand: Higher temperatures increase evaporation and plant transpiration, causing a reduction in soil moisture available for the plants to grow. This also leads to the plant closing their stomata to avoid extracting too much water from the soil. This then reduces CO2 uptake and stimulates root growth at the expense of the part of the plant that is above the ground.
When we started the study it was clear that crop models participating in ISIMIP did not account for direct temperature-induced crop damage. The effect is also assumed to occur at even higher temperatures than the 30°C threshold found by Schlenker and Roberts (2009). In addition, there already was one study supporting the hypothesis that the observed yields reduction could be caused by water deficits induced by high temperatures. In contrast to the direct damage to plant tissues at very high temperatures the water deficit effect should be included in the models. So there was some hope that the ISIMIP models would also show a drop in yields at high temperatures. However, it was not clear whether the simulated effects are as strong as the observed effects. The new statistical approach provided an ideal test case for our models.
How can you estimate the yield response to exporure to different temperature levels when we only know yields at the end of the growing season?
Well, basically you cannot do this when you have only one observation of yield decline. Then low yields at the end of the growing season can be caused by many different factors. However, Schlenker and Roberts (2009) showed that when you have enough observations (many years across a large number of regions) it is possible. The final yield is the product of all factors that influence crop growth, directly or indirectly. So if crop yield is lower on average across many observations when there is a lot of exposure to high temperatures, we can assume that the high temperatures are associated with a yield decline.
For the US there are enough observations as there is exceptionally good documentation of crop yields from 1950 to today at the county level (see Figure 1).
Agricultural land-use for major crops in US counties
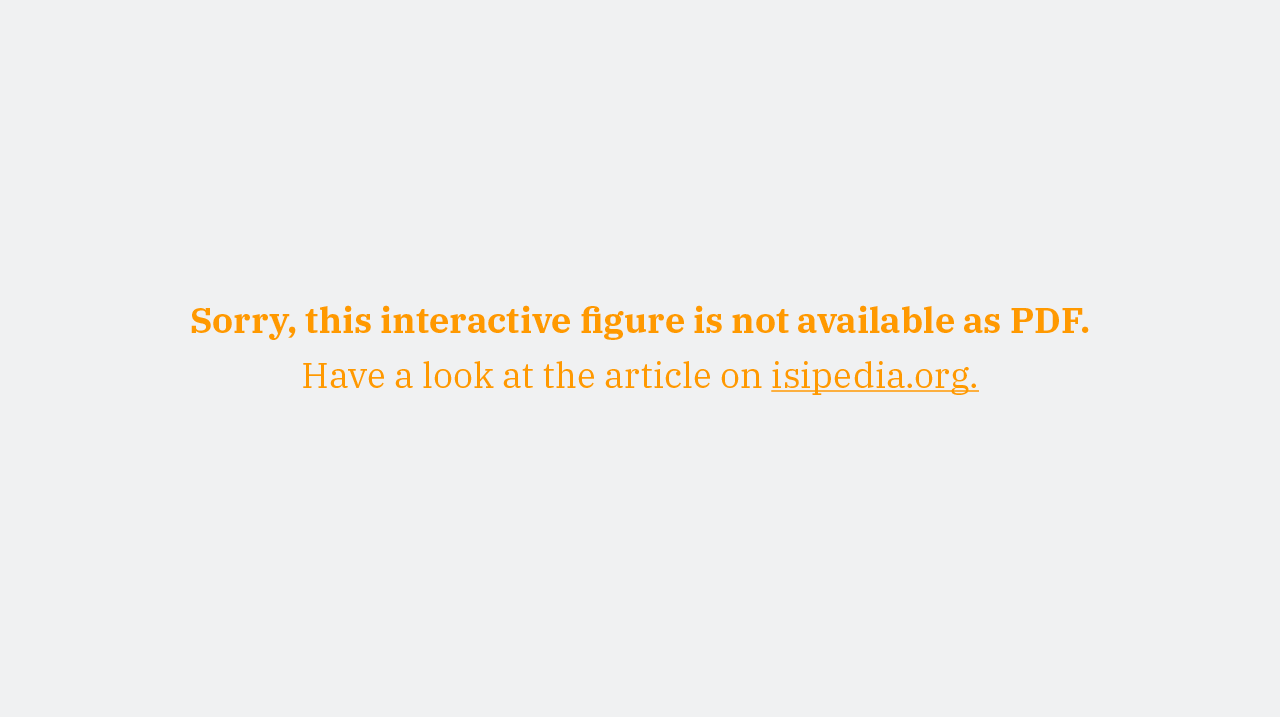
The percentage of land use for irrigated and rainfed maize, wheat and soy per US county. Click on the buttons to show the various options.
FIG 1 / Adapted from Schauberger et al. (2017).
So why not use the same statistical approach they used but apply it to both observed and simulated yields? In doing so, we can test whether the yield reduction due to exposure to the considered temperature intervals is the same for both observations and simulations. We proceeded to do so for crop simulations of 9 crop models (EPIC-Boku, EPIC-IIASA, GEPIC, LPJ-GUESS, LPJmL, ORCHIDEE-crop, pAPSIM, pDSSAT and PEGASUS) generating historical simulations forced by observed weather observations within ISIMIP2a. As the simulations only covered the time period from 1980-2010 (1980-2008 for wheat) we also restricted the observed data to this period. In addition to the individual crop models specific yields simulated for each county and year we also consider results of a ‘median model’ by always calculating the median yield of the 9 individual yields generated by each crop model to provide a summary across the entire model ensemble.
While the models simulate maize and soy also considered by Schlenker and Roberts (2009) they do not provide cotton simulations. Instead we included wheat in our analysis.
In addition, we separate mostly rainfed counties (more than 90% of the considered areas where the crop is grown) from mostly irrigated counties (more than 75% of the areas used to grow the considered crop irrigated) and also assume that crops are purely rainfed in the first group and fully irrigated in the second case. The separation of the two cases is important as we expected that water constraints may play an important role in the observed drop in yields (see arguments above).
What did we find?
Yields under rainfed conditions (click on rainfed in the Figure)
First of all our analysis of reported rainfed maize and soy yields revealed patterns of ‘efficiencies’ of exposure to different temperature intervals that are very similar to the ones found by Schlenker and colleagues although we did not use data before 1980 and added the year from 2005-2010 (2005-2008 for wheat). Yield for rainfed maize and soybean significantly declines when daily temperatures reach levels above ∼30 °C. Temperatures between 33°C and 36°C have been especially harmful: each additional day within this temperature range reduced yield of maize and soybean by ~2-4%. For wheat (we only consider winter wheat in our analysis) such a reduction of yields at high temperatures cannot be found.
And yes, the rainfed yields described by the median model match the patterns of yield contributions of one day spent at the temperature range indicated at the x-axis. In particular the reduction at high temperature found for maize and soy is reproduced by the median model. Observations and simulations also agree on not finding a similar drop for wheat. This means that the observations can be explained by our knowledge about the relation between weather conditions and yields as implemented in our models even without representing a specific mechanism that directly results in plant damage at high temperatures. While the median crop model simulates the crop yield declines at high temperatures well, individual models do not necessarily reproduce the observed pattern of yield responses (click through results for individual model results).
Temperature dependence of yields under rainfed or irrigated conditions
Model irrigation is
The displayed simulation is from model .
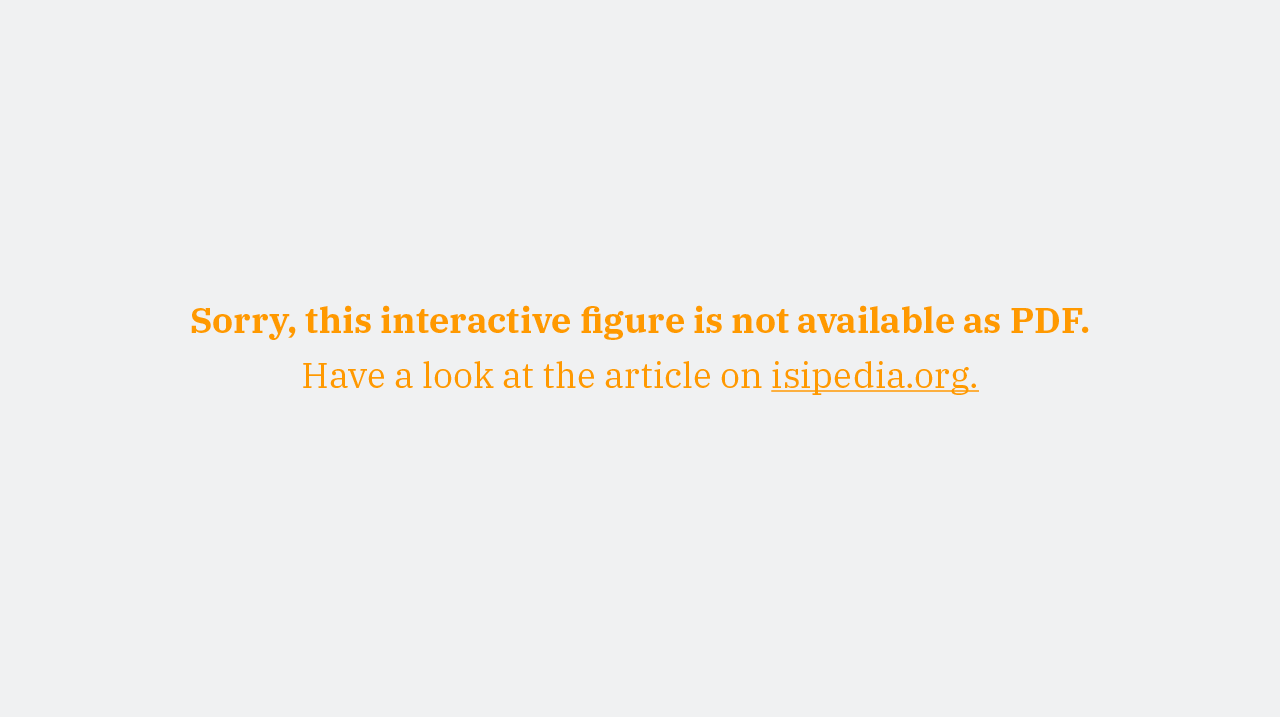
FIG 2 / Adapted from Schauberger et al. (2017).
Yields under irrigated conditions (click on irrigated in the Figure)
The negative impact on yield at high temperatures above 30°C is only minor or completely disappears in the simulations when considering yields under irrigation for all crop types, supporting the hypothesis by Lobell et al. (2013) that it is mainly the reduced availability of soil moisture that drives these yield declines. Our results indicate that the selected ensemble of process-based crop models successfully simulates yield declines at high temperatures. Other explaining factors, such as direct damage to the plant tissue, impaired flowering and oxidative stress are not included in most crop models, also pointing to reasons for the decline other than direct damages.
A detrimental effect is still observed for rainfed (but not irrigated) maize at temperatures above 39°C, but due to the small amount of data points, the confidence in this result is low.
As expected, irrigated areas also show a much higher evapotranspiration due to the increased availability of water, and a significantly higher biomass accumulation. All crop models simulate a shorter growing season for irrigated maize and soybean, with higher average temperatures. Only some models simulate a shorter growing season for wheat due to vernalization effects.
Direct damage to plant tissues is expected to play a larger role only at temperatures above 35°C or more, indicating that the selected crop models should not - in their current state - be used to simulate yields at extremely high temperatures above 35°C.
Main open issues
While the median of the model ensemble represents the observed data quite well, the results of the individual models can be quite different from the observed data. It will be necessary to understand the reasons for some of the mismatches between the simulated and observed yield responses, requiring further study into the details of the models.
There are differences between the crop models, both in the type of processes they include and the way these processes are simulated. For example, only the crop model PEGASUS simulates impaired flowering, while most but not all models simulate enhanced root growth under higher temperatures to tap further water resources. Other processes, while included in all models, are implemented and calibrated differently leading to different results.
One additional potential reason for the mismatches between observed and simulated data could be the differences between the actual growing seasons and the ones assumed in the model. Researchers have shown that spatially varying growing seasons are important to reproduce fluctuations in crop yields Jägermeyr and Frieler (2018). In contrast, we use fixed growing seasons for the whole United States. Instead, growing seasons start and end change from year to year and from location to location. This could lead to an inappropriate consideration of heat days as farmers may change harvest and growth dates in response to temperature changes and predictions. Yet for the purpose of our study on a large geographic scale this assumption was appropriate, evidenced by limited sensitivity of results to season assumptions.
Acknowledgments
We thank Frank Wechsung from PIK Potsdam for insightful discussions.
This article was written in collaboration with the Editorial team of ISIpedia project.
References
Cover image: Jean Wimmerlin
Affiliations
1 Climate Impacts and Vulnerabilities, Potsdam Institute for Climate Impact Research (PIK), 14473 Potsdam, Germany
2 Department of Agronomy, Iowa State University, Ames, Iowa 50011, USA
3 Institute of Meteorology and Climate Research-Atmospheric Environmental Research (IMK-IFU), Karlsruhe Institute of Technology, 82467 Garmisch-Partenkirchen, Germany
4 International Institute for Applied Systems Analysis, Ecosystem Services and Management Program, Schlossplatz 1, A-2361 Laxenburg, Austria
5 Department of Soil Science, Faculty of Natural Sciences, Comenius University in Bratislava, 84215 Bratislava, Slovak Republic
6 Laboratoire des Sciences du Climat et de l'Environnement, Institut Pierre-Simon Laplace (IPSL), 91191 Gif sur Yvette, France
7 University of Chicago and ANL Computation Institute, Chicago, Illinois 60637, USA
8 Department of Geography, Ludwig Maximilian University, 80333 Munich, Germany
9 School of Geography, Earth & Environmental Science and Birmingham Institute of Forest Research, University of Birmingham, Birmingham, B15 2TT, UK
10 University of Natural Resources and Life Sciences, Vienna, Feistmantelstrasse 4, 1180 Vienna, Austria
11 Laboratoire de Météorologie Dynamique, Institute Pierre-Simon Laplace, 95005 Paris, France
12 Sino-French Institute of Earth System Sciences, College of Urban and Environmental Sciences, Peking University, Beijing, 100871, China
13 School of International and Public Affairs, Columbia University, New York, New York, 10027, USA