The global loss of biodiversity has increased rapidly over the last 50 years. Biodiversity is essential for ecosystem functioning and the provisioning of ecosystem services and thus ultimately affects human well-being. One major threat to biodiversity is climate change. Reducing global warming is therefore vital to reduce the threat to biodiversity. The Paris Agreement from 2015, calls not only for a reduction of emissions to hold the increase in global average temperature to well below 2 °C but to also pursue efforts to limit global warming to 1.5 °C. One of the proposed measures to stay on the 1.5 pathway is to promote the development of renewable energies, including biofuels. This would require a substantial expansion of existing biofuel cropland. Unfortunately, such large scale land-use changes are accompanied by various negative impacts including potential biodiversity loss. So do these measures not destroy the very thing we want to protect?
Therefore, we wanted to investigate the impacts of the expansion of bioenergy cropland on biodiversity under a low-warming scenario and compare them to a high-warming scenario without bioenergy cropland expansion. A better understanding of the potential impacts of climate and land-use change under different warming scenarios could support strategic decisions on the measures that will be taken to reduce global emissions.
So how do we estimate the effect of climate change and land-use change on biodiversity?
Species distribution models
To make our assessments about the impacts of climate and land-use change on biodiversity, we used species distribution models. These models link the occurrence of a species to certain environmental conditions such as climate variables. These links can then be used to project where a species is likely to occur based on the environmental suitability. For example, when the temperature is projected to increase, a species model might predict that a certain species with a lower temperature tolerance goes extinct in an area, while another species with a higher temperature tolerance might thrive.
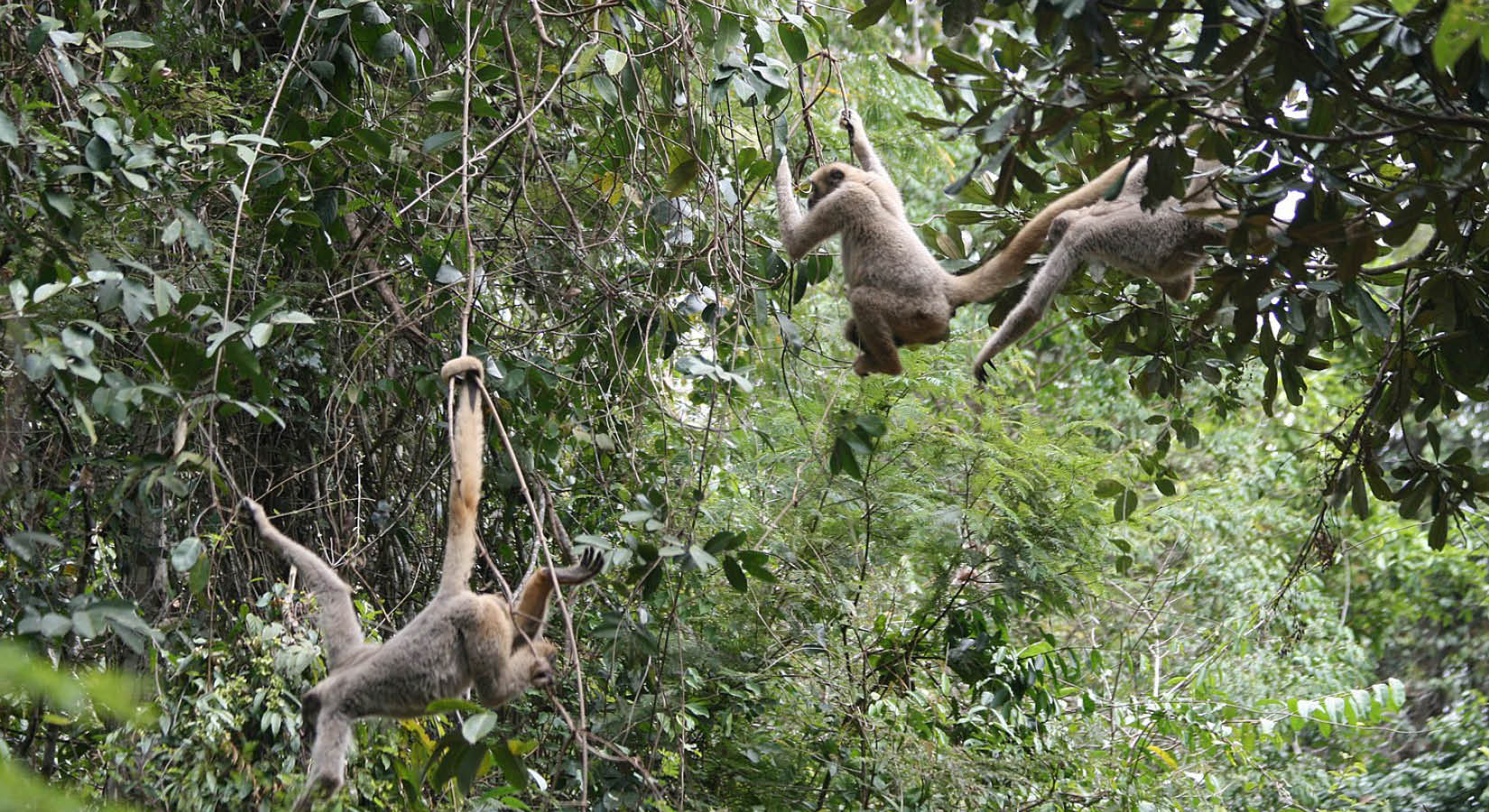
The Northern Muriqui Monkey lives in the Atlantic Forest, an area projected to face high level climate and land-use change threats. It is listed on the IUCN Red List of Threatened Species 2021.
FIG 1 / Image Source: Kenny Ross
Species are not only responding to climate change by reducing their ranges (becoming locally or globally extinct), but also by shifting their distributions. A species might go locally extinct but could potentially migrate to an area with a more suitable climate. If a species is able to migrate under climate change depends largely on its natal dispersal ability. The natal dispersal ability is defined as the average distance between the birth site and the breeding site of a species and varies widely across species. Plants, for instance, have a much lower capacity to migrate and it takes them, eventually, much longer in comparison to large mammals, which have a faster response and greater ability to move and shift environments. Therefore the species distribution models used here also include a measure of the potential dispersal ability. In our study we use several scenarios of potential dispersal to estimate the distributions of three vertebrate taxa, mammals, birds and amphibians, under different warming scenarios. In a no-dispersal scenario we assume that species stay in place when the climate changes (i.e., worst case scenario). Therefore, if the climate is not suitable anymore, the species goes extinct in that location. In a dispersal scenario, the species can migrate to a more favorable location nearby their original range. age
Collecting data on species, climate and land use change
To run these species distribution models for future climate we first require information about the current suitable conditions for individual species. We derived freely available expert range maps for the three vertebrate taxa (6,380 amphibians, 5,275 terrestrial mammals and 9,882 terrestrial birds) from IUCN (Conservation of Nature and Resources 2016) and BirdLife International and Nature Serve (International and NatureServe 2015). Based on these maps we derive the range of climatic conditions a species can persist under.
In addition, we require information about the future climate. Here, we used an ensemble of 4 global climate models (MIROC5, GFDL-ESM2M, HadGEM2-ES and IPSL-CM5A-LR) under a high warming scenario (RCP6.0), and a low warming scenario (RCP2.6).
Additionally we use the projections of future land-use change which are provided by ISIMIP2b. The land-use projections stem from the MAgPIE and REMIND-Magpie model and consider population growth and economic development for the two warming scenarios (RCP2.6 and RCP6.0). To reach the low warming scenario (RCP2.6), the expansion of land for bioenergy crops is currently an assumption in most projections. In addition to bioenergy croplands, we also include the projections of the expansion of other crops and pastures. For the majority of species, a conversion of natural habitat to anthropogenic land-use implies a degradation in the suitability of the area for the species, although there are exceptions. Therefore, if the anthropogenic land-use in a given grid cell increases under the future projection we include this as a decrease in the suitability of the area for the species present in the cell. The projected land-use change is overlaid with the projected impacts of climate change on biodiversity, to identify areas where species are affected by none of the two threats, a single threat (climate change or land-use change) or both threats.
What are the results of our projections?
The impacts of climate change only are projected to be stronger under the high warming scenario compared to the low warming scenario. Looking at the overall numbers of terrestrial vertebrate species impacted by climate under the two different emission scenarios, we found that, of the 15,448 species included in the species distribution models, a slightly higher number of species will be exposed to climate change alone under the high emission scenario (RCP6.0, 14,989 ± 142) compared to the low emission scenario (RCP2.6, 14,855 ± 129).
Climate change is a threat for global vertebrate diversity
Reduction in number of species due to climate change in a scenario climate mitigation:
The map shows the change in the number of species by the year compared to 1995, under the sole effect of climate change (no land-use change is considered here). A negative number indicates a reduction, and a positive number indicates an augmentation in the number of species, from a total of species investigated worldwide in . Calculations assume .
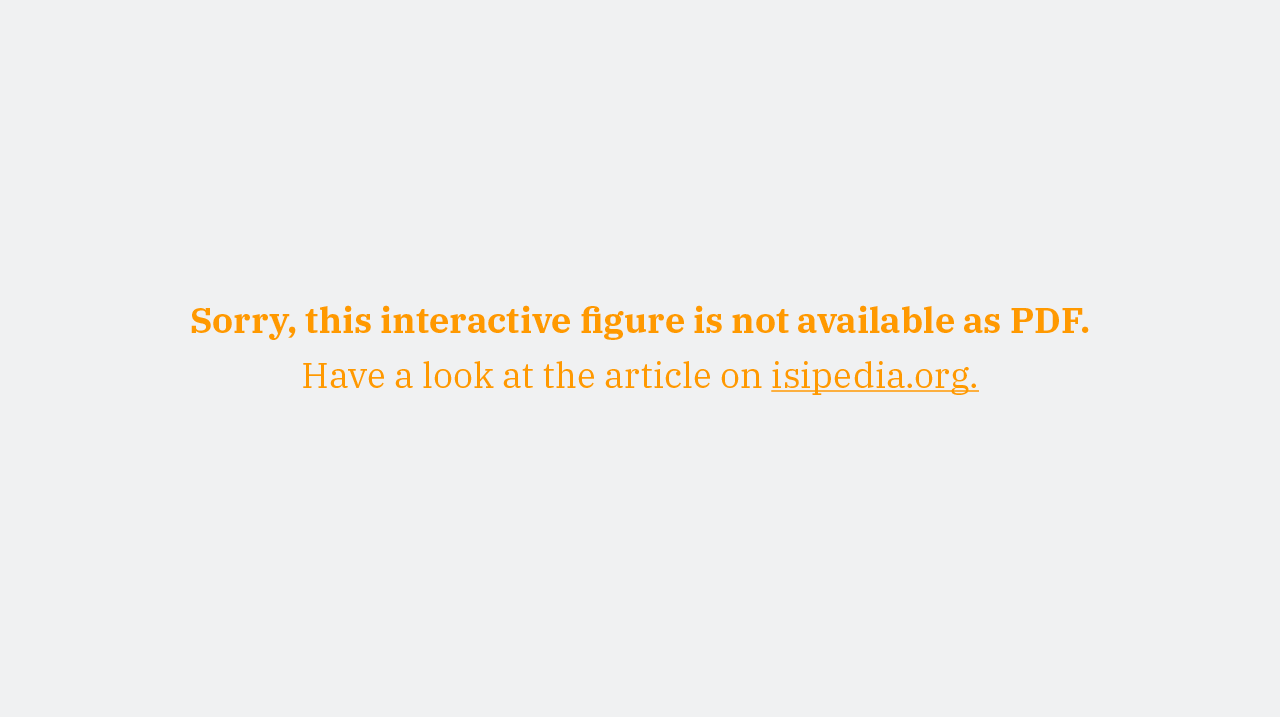
FIG 3 / Adapted from Hof et al. (2018).
The spatial pattern of the changes in species richness are similar between the two emission scenarios. In both scenarios, areas of highest projected climate-driven changes in species richness are in South America and in New Guinea, reaching potential losses of up to 150 species of investigated species in the area. While areas with potential losses of between 20 and 70 species are scattered across all continents. Figure 3 shows the impacts of climate change on species richness globally under the high warming scenario and the low warming scenario.
Focussing on the impacts of land-use change alone, we found a much larger number of species to be potentially affected by bioenergy cropland expansion alone under the low warming scenario (RCP2.6, 10,346 ± 370) compared to the high warming scenario (RCP6.0, 2,054 ± 279). These expansions are projected to be especially widespread across Europe, western North America and Asia.
Global vertebrate diversity threats with and without climate mitigation
Global vertebrate diversity threats climate mitigation:
orThe map shows the distrubution of threats by the year , for , assuming .
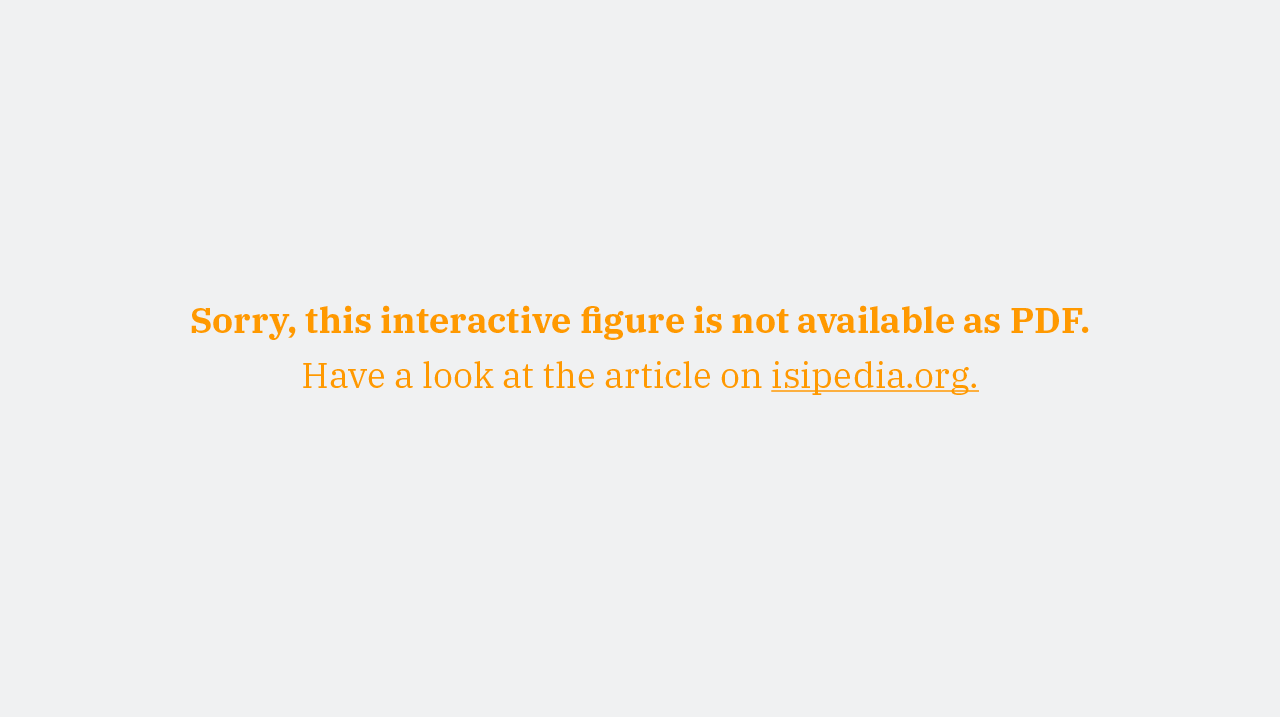
FIG 4 / Adapted from Hof et al. (2018).
When looking at the effects of both climate threat and land-use change threat together (including climate only, land-use only and combined impacts), a slightly larger number of species will potentially be affected under the low warming scenario (RCP2.6, 15,309 ± 92) than under the high warming scenario (RCP6.0, 14,994 ± 140). Overall, most areas with high levels of projected combined climate and land-use change threats are in the tropics: Southwestern South America (mainly the Pampas, Atlantic Forest, and Cerrado regions), Central America, large parts of tropical Africa and south and southeast Asia. Figure 4 shows the distribution and extent of the different threats (i.e., climate change and various components of land use change).
In both warming scenarios about 36% of the terrestrial area is projected to undergo a reduction in species richness either through climate change, land-use change or a combination of both threats. As expected, the impacts of climate change are much more pronounced under the high warming scenario (RCP6.0) while the extent of impacts due to land use change is much higher under the low warming scenario. These results suggest that from a biodiversity perspective pursuing the 1.5 °C goal is highly desirable. Nevertheless, they also highlight the urgency to carefully consider potential impacts on biodiversity when expanding bioenergy cropland.
What are the limitations of our projections?
While the study is sufficiently robust to outline broad geographic and numeric trends for entire taxa, some caveats need to be considered. Especially those that are inevitably inherent to large-scale studies that rely on simplifications and complexity reductions.
First, our study relies on expert-based range maps as input data for the species distribution models. Both the usefulness of range maps as well as the appropriate spatial resolution for macroecological analyses are a matter of debate. For this study we gridded the range maps onto a 0.5° x 0.5° degree resolution, resulting in cell sizes of more than 3000 km2 at the equator, which is relatively coarse. This can result in mismatches between actual species occurrences and the microclimate and habitat these species occur in and the coarse averages of the climatic conditions and land-use types included in the modelling. Because of these simplified assumptions the study results can be used to outline broad trends, but should not be used for species- or locality- specific interpretations.
We assumed that an expansion of cropland, bioenergy cropland or pasture at the expense of more natural vegetation has a negative impact on the species richness. While it is likely that this holds true in most cases, and in particular for monoculture croplands with intensive management, this is clearly not the case for all land management systems. Even bioenergy cropland can under certain circumstances increase biodiversity and non-yield ecosystem services, such as soil protection (Diacon-Bolli et al. 2012). Global scenarios of the impacts of land use change on biodiversity based on a more detailed representation of different land use systems do not exist.
The applied species distribution models solely represent the potential impacts of changes in climatic conditions on species distributions. Species distribution models do not include any biological interactions, which could change species responses to climate change.
Finally, the ability to integrate land-use and climate change into a joint modelling framework is still limited. While promising approaches to incorporate species specific habitat preference into these types of models have been proposed (Rondinini et al. 2011; Methorst et al. 2017), such efforts still remain challenging at the spatial scale and the taxonomic extent that this study was conducted at (Kok et al. 2017).
Acknowledgements
We thank the Inter-Sectoral Impact Model Intercomparison Project (ISIMIP) and especially L. Warszawski, C. Reyer, K. Frieler, and S. Ostberg, as well as everyone else involved in ISIMIP climate and land-use data, for their support and making the data available. This work was supported by the German Federal Ministry of Education and Research. We also acknowledge support by the Bavarian Ministry of Science via the Bavarian Climate Research Network (bayklif) (C.H., M.F.B., and E.K.E.) and by the German Young Academy (Die Junge Akademie) (C.H.).
This summary was written in collaboration with the Editorial team of the ISIpedia project.
References
Affiliations
1 Senckenberg Biodiversity and Climate Research Centre (BiK-F), 60325 Frankfurt, Germany
2 Department of Biosciences, Durham University, DH1 3LE Durham, United Kingdom
3 Terrestrial Ecology Research Group, Technical University of Munich, 85354 Freising, Germany
4 Department of Physical Geography, Geosciences, Johann Wolfgang Goethe University of Frankfurt, 60438 Frankfurt am Main, Germany
5 Department of Biological Sciences, Institute for Ecology, Evolution and Diversity, Johann Wolfgang Goethe University of Frankfurt, 60438 Frankfurt, Germany