Our short answer is yes. This article summarises how we arrived at this conclusion and lets you explore the long answer. We explain how we did our research, which data we used, how those data were produced and how they were analysed. First we outline what was already known when we started working on this question. At the end, we discuss some of the limitations of our results. This article is about our motivation, methodology and results at the global and national level. In the column to the left you can access more detailed results for individual countries.
Why did we expect climate change to change the exposure to droughts?
A drought occurs if there is less water available than usual. Both a lower than usual supply of water and a higher than usual demand for water can result in a drought. Global warming has an impact on both sides of the water budget. First, it has an impact on the supply side because it changes precipitation patterns around the world. It also has an impact on the demand side because warmer air can hold more water vapour and hence global warming increases the evaporative demand of the atmosphere. In conclusion we expected that global warming has an impact on the water budget and the occurrence of droughts.
This expectation was supported by a range of studies before we started our analysis. At the global level, most of those studies (e.g., Dai (2013), Prudhomme et al. (2014), Lehner et al. (2017)) found that global warming increases the supply of water through increases in precipitation but that it increases the evaporative demand of the atmosphere even more. Hence they projected an increase in the occurrence of droughts in response to global warming, with the magnitude and spatial pattern of that increase varying from study to study.
With our analysis we wanted to verify the finding of an increase in drought occurrence at the global level and learn more about the spatial pattern of that increase. In addition we wanted to quantify exactly how much more land and how many more people are annually exposed to droughts per degree of global warming.
How did we project land and population exposure to droughts?
In order to arrive at our projections, we first needed to define what we consider a drought event, how we measure the exposure to such an event, and how we simulate these events.
There are several types of droughts. For example, a meteorological drought occurs when precipitation is abnormally low for a longer time period, and a hydrological drought occurs when river discharge is abnormally low for a longer time period. Here, we decided to consider droughts that occur in the form of soil moisture deficits (i.e., agricultural drought) because those droughts are expected to have a direct impact on plant growth, both for natural vegetation and agricultural crops. More specifically, we defined that a drought occurs if the monthly mean rootzone soil moisture is much lower than usual for at least 7 consecutive months. Here, “much lower than usual” means that soil moisture deficits of that magnitude only occur 5 times in 200 years under pre-industrial climate conditions.
Land exposure to droughts was measured by the land area under drought conditions and population exposure to droughts was measured by the rural population living in that area. For our population exposure projections we used the global population distribution of the year 2005. By using this fixed distribution we ensured that our projections represent only climate change related impacts, not those related to future population change.
In order to simulate soil moisture conditions, we used climate models and hydrological models. Climate projections were made with four global climate models (i.e., GFDL-ESM2M, IPSL-CM5A-LR, HadGEM2-ES, MIROC5). Those were then used to make hydrological projections with eight global hydrological models. Finally, droughts were identified from the soil moisture output of these hydrological projections.
In the next section, we present results of our projections both in the form of time series and at different levels of global warming relative to pre-industrial conditions. The results at different warming levels were derived by pooling results from all years with a global mean temperature sufficiently close to the respective warming level.
For a full methodological description with more details please see our paper Lange et al. (2020).
What are the results of our projections?
Compared to pre-industrial conditions, global warming has already reached 1°C today. For this warming level, we simulate that 2.7% of the global land area (excluding Greenland and Antarctica) and 0.77% of the global population is exposed to at least one drought per year. For 2°C global warming, we project these numbers to increase to 4.44% of the global land area and 1.32% of the global population. These are increases by a factor of 1.64 and 1.71, respectively. The number of people annually exposed to droughts is projected to increase from 58.44 million at 1°C to 100.07 million at 2°C global warming, again, in terms of the 2005 global population.
In Figure 1 you can explore those projections at the global level. The toggles land/population and global warming/time allow you to change what is represented by which axis of the line plot. For example, you can view how land exposure to droughts varies with global warming and how population exposure to droughts changes over time for different climate change scenarios (RCP2.6 and RCP6.0).
By default, Figure 1 shows the median of the results obtained with all climate model–hydrological model combinations. Lines and dots represent the exposure projected for an average year. The shading represents how much the exposure typically deviates from that average as it varies from year to year. To see results for an individual model combination, use the drop-down menus for model selection. You will find that depending on which climate model you select, exposure variation with global warming is shown for different ranges of global warming. This is because different climate models reach different levels of global warming towards the end of the 21st century under the two future climate change scenarios used in our study. The highest warming level reached by all climate models is 2°C. That is why median results over all climate models are only shown up to that warming level.
Explore how land and population exposure to droughts vary with global warming and time at the global and national level
Show exposure of
Show how exposure varies with
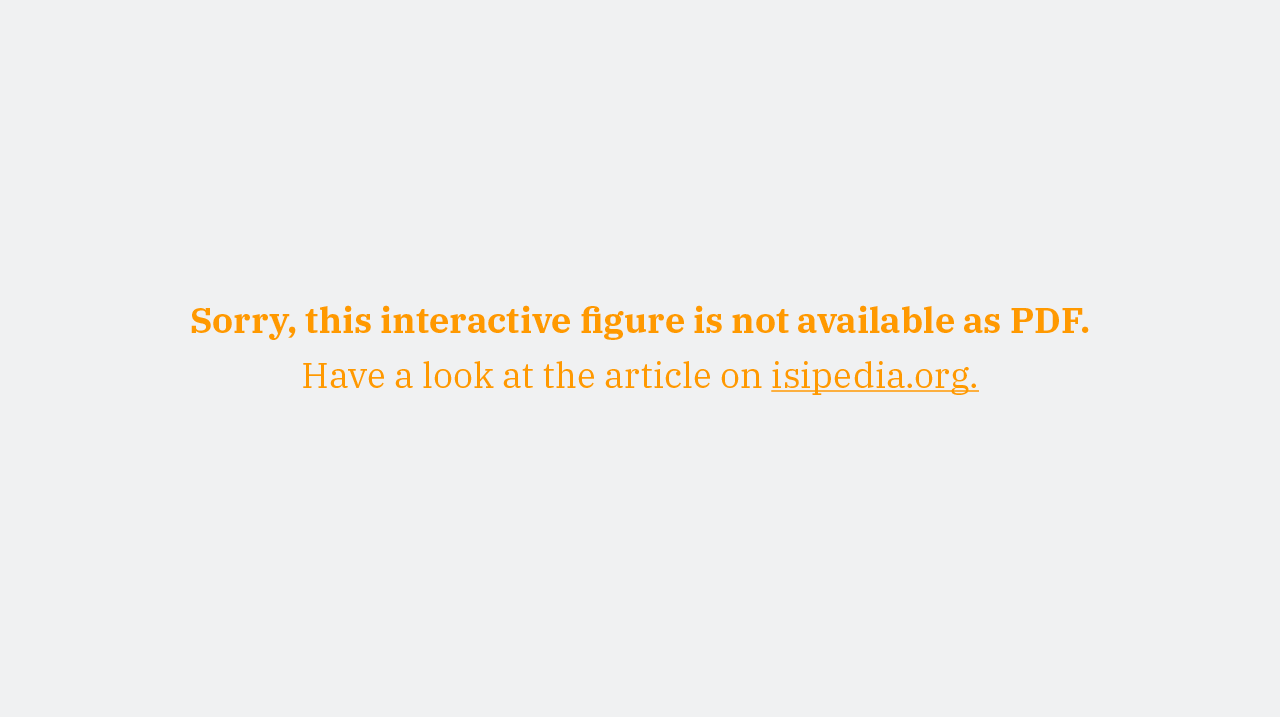
Click on any dot to see a disaggregation of the global results to the national level. Compare results obtained with different models by selecting All in the drop-down menus or by clicking on their headers. Use the toggles to change what is represented by the axes of the line plot. The NDC line represents the expected global warming level for the given year assuming the mean of the unconditional worst, unconditional best, and conditional NDC pathways. See this article for more info. Use the Download Data button to get numeric results for all model combinations.
FIG 1 / Adapted from Lange et al. (2020).
What you can learn from Figure 1 is that in global terms the exposure to droughts increases as the world warms. Higher greenhouse gas emissions under RCP6.0 compared to RCP2.6 lead to stronger global warming, which results in more droughts. The exact magnitude of the increase in droughts in response to global warming is uncertain though. This uncertainty is represented by the range of results obtained with different model combinations.
If you click on a dot in Figure 1 you will see results at the national level that correspond to the global results represented by the dot. You will find that exposure to droughts varies considerably from country to country. You can explore these differences further in Figure 2, which shows the multi-model median exposure to droughts in an average year at 2°C global warming for different countries. Next to that, Figure 2 ranks countries by those exposure values. According to that ranking, the largest exposure to droughts in terms of the national land area percentage annually exposed at 2°C global warming is projected for West Bank and Gaza (1), Morocco (2), Malta (3), Israel (4), Algeria (5), Libya (6), Lebanon (7), Syrian Arab Republic (8), Western Sahara (9) and Cyprus (10).
Explore how land and population exposure to droughts at 2°C global warming vary from country to country
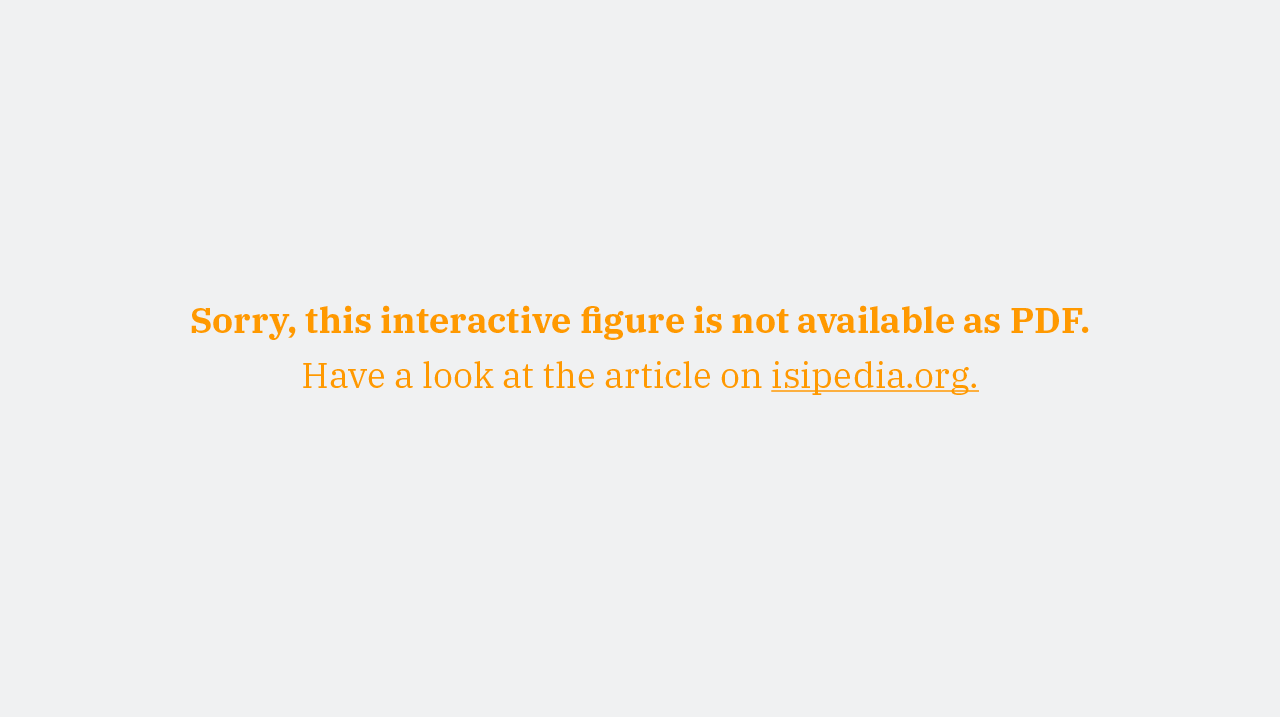
Use the toggles to switch from a country ranking by exposure to annual average exposure values and from land exposure to population exposure. Use the Download Data button to get numeric results for the current view.
FIG 2 / Adapted from Lange et al. (2020).
What are the limitations of our projections?
The limitations of our projections are related to our drought definition, our measure of exposure and to the way we simulated droughts.
The drought definition used in our study indicates a drought if the soil is much drier than usual under pre-industrial conditions. That makes sense for global drought projections because it facilitates the comparison of drought conditions across time and space. However, it does not reflect how much water is available in absolute terms. Therefore, exposure to drought as defined in our study should not be confused with exposure to water scarcity, which relates human water demand to environmental water supply. Moreover, since we considered only droughts that last for at least seven consecutive months our analysis provides no indication with respect to short-term droughts under climate change.
Furthermore, we assumed that only rural populations are exposed to droughts. This was based on the assumption that people living and working in the countryside depend more on soil moisture than urban dwellers. This clear cut separation clearly is a simplification of reality since prolonged droughts affect urban populations too. In addition, our exposure projections do not consider future population change. While this was done to isolate the pure effect of climate change it is certainly not realistic. People moving into or out of drought-prone areas as well as different population growth rates in different world regions will influence future population exposure to droughts.
It is also important to keep in mind that the models used here are simplifications of reality. Since different models use different simplifications, the results shown above are model-dependent. For droughts as defined in our study, the greatest contribution to this modelling uncertainty comes from the global hydrological models. This is because rootzone soil moisture is notoriously difficult to both simulate and measure. In our models, it is calculated as the difference between the amount of water coming in as rain or snow, and the amount of water going out, through evaporation from the surface,plant transpiration, as runoff, or through percolation of water towards the deeper groundwater layers. All of those processes are represented differently in the different models.
The arguably most uncertain of those processes is evapotranspiration. While some models calculate it directly, most models first calculate what is called potential evapotranspiration as an upper limit to actual evapotranspiration. Unfortunately, there are various approaches to calculate potential evapotranspiration and it is currently unclear which of those is most appropriate for future projections (Milly and Dunne 2017; Wartenburger et al. 2018). Another challenge lies in the representation of how vegetation cover affects actual evapotranspiration, a relationship that is subject to change under rising temperature and CO2 levels. For example, the effect of CO2 fertilization – the phenomenon through which photosynthesis, hence plant growth, should be enhanced in a CO2-richer atmosphere – is not represented in most of our global hydrological models. In reality, plants need less water to assimilate the same amount of carbon in a CO2-richer atmosphere. This effect alone would lead to a decrease of evapotranspiration. Yet the more efficient carbon assimilation lets plants grow better, which leads to larger or more plants. This effect alone would lead to an increase of evapotranspiration. The overall CO2 fertilization effect on evapotranspiration, soil moisture conditions and drought risk is uncertain (Prudhomme et al. 2014; Döll et al. 2016; Kuzyakov et al. 2019).
Our drought projections also diverge because the soil column is represented differently in different models. In one model the soil column is represented by 15 layers down to a depth of 42 meter. In another case it is represented by a single soil layer with a depth of 1 meter. In addition, the models make different assumptions about root depths. As a result, the definition of the root zone varies across models.
Finally, our drought projections do consider future changes in land use, land cover, and irrigation. Even in the historical period our simulations only account for water abstractions (for irrigation, livestock, industry and domestic use) from groundwater, surface waters and reservoirs while other processes such as inter-basin water transfers and a more realistic reservoir management are also important factors. Including these drivers into our simulation would greatly improve the simulations. That this is not done is mainly due to missing knowledge about them.
References
Cover image: ICARDA
Affiliations
1 Potsdam Institute for Climate Impact Research, Member of the Leibniz Association, Potsdam, Germany
2 Vrije Universiteit Amsterdam, The Netherlands
3 Goethe University Frankfurt, Germany